
COGNITIVE NEUROSCIENCE
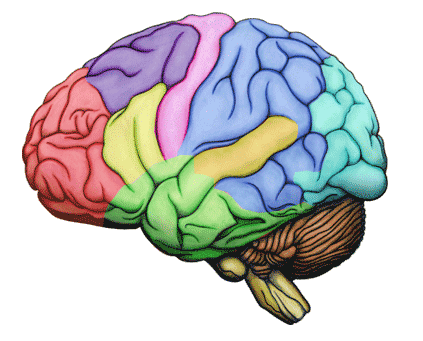
Brain Research Methods
Tip: Click on the arrows to enlarge the screen
MRI Video
Brain Research Methods Presentation
Tip: Click on the box to enlarge the screen or go to the Video Files section
Brain Research Methods
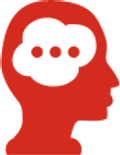

BRAIN VIDEOS



Brain Imaging Techniques

A number of techniques are available to investigate the question of how and where in the brain particular perceptual and cognitive processes occur. Tasks or tests can be devised that place varying levels of demand on the cognitive, sensory or motor capacities of the participant being tested. Performance of these tasks is then correlated with physiological measurements, and on the basis of these results, we may go on to ascribe functions to areas of the brain.
Whilst there is a growing fascination with imaging techniques in the popular media (e.g. ‘This is your brain on politics’), it’s important to bear in mind that each technique has limitations that often don’t get picked up on by newspaper editors who themselves have little-to-no experience using them. The most often overlooked limitation is the issue of ‘reverse inference’ – just because cognition X (e.g. using one’s memory) is associated with brain activation Y (e.g. activation in the prefrontal cortex), that doesn’t mean that if a participant displays activation Y, they are necessary engaging in cognition X.
Below we highlight some key neuropsychological and neuroscientific techniques, and a few of their limitations. There are also a number of great blogs that deal with issues relating to neuroscience, particularly in the popular press.
Testing brain damaged subjects (Neuropsychology)
Neuropsychology, the precursor to modern neuroscience, allowed us to learn a great deal about brain function by examining people with specific, known brain injuries. Cognitive changes are often reported in people who have suffered some kind of brain injury. The damaged areas are a good indicator of the brain regions that are important to the cognitive function that has been changed by the injury.
One of the most striking examples of this form of neuropsychology was carried out on a railroad construction worker, Phineas Gage. Following an explosives accident, Gage survived a cylindrical iron rod being driven completely through his head. This destroyed his left frontal lobe and changed his personality so much that his friends described him as “no longer Gage.” Whilst little formal psychological testing was carried on Gage, anecdotes about changes in his behaviour are used to illustrate the role of the frontal lobes in healthy decision-making and emotion.
Other notable cases include patients HM and Tan who have informed modern psychological understanding of the brain involvement in memory and speech production.
Problems: Specific deficits in processing are rarely found without the occurrence of other deficits. Psychological observations are usually only ever made after the event and therefore lack adequate experimental control.
Lesion studies (Neuropsychology)
The removal of part of the brain - comparison is made between performance before and after the lesion and consequent deficits are noted. This branch of neuropsychology sounds brutal, but is often carried out when brain surgery is required (e.g. to remove parts of the brain where epileptic seizures are known to originate). Lesion studies have informed our understanding of which brain regions can be removed with minimal consequence for the patient,
Problems: In practice, it is difficult to make a brain lesion that entirely removes one part of the brain whilst leaving the rest entirely intact. Lesions may damage other systems which happen to pass through the lesion site.
Stimulation (Neuropsychology/Neuroscience
This involves feeding a signal (chemical or electrical) into some part of a neural circuit and measuring its consequences. Invasive stimulation involves surgery and is often carried out as part of a procedure to assess which regions of the brain it might be least disruptive to lesion. For example, it is very important to leave brain regions associated with language processing intact so that surgery does not totally impair a person’s ability to communicate.
Non-invasive stimulation in the form of transcranial magnetic stimulation (TMS) is a relatively recent development in the experimental neurosciences. A coil is held up to the head of healthy participants, positioned directly over the part of the brain which is intended to be temporarily stimulated, and causes neurons on the surface of the brain (nearest the coil) to discharge, effectively temporarily lesioning that brain region. This technique allows experimenters to conduct lesion-like studies on large number of participants.
Problems: Difficulties of invasive stimulation involve delivering stimulation at an intensity that mirrors the level of activity that spontaneously occurs in the brain and determining which structures have been affected by the stimulation. Non-invasive stimulation is prone to the same difficulties as lesion studies in localising the temporarily induced lesions. Limitations of the magnetic field also mean that only areas on the surface of the brain can be stimulated and care must be taken to avoid stimulation in regions near muscle that might twitch as a result of their own stimulation.
Single-cell recording (Neuroscience)
Microelectrode recordings indicate specific neuronal networks dedicated to processing particular stimuli. (e.g. bars of a certain orientation, movement in a particular direction, particular objects like faces). For much of the 1950's physiologists probed the visual cortex using this technique.
CT Scan (Neuroscience)
This technique takes advantage of the fact that X-rays reflect the relative density of the tissue through which they pass. If a narrow X-ray beam is passed through the same object at many different angles, it is possible to use computational techniques to construct a visual image of the brain.
Problems: Prolonged or repeated exposure to ionising radiation can cause tissue damage. Thus, as with x-rays, CT scans are used sparingly. Additionally, only structural information about the brain can be gathered. This is perfect for identifying problematic brain tissue, but gives us little insight into how the brain functions during cognition.
PET (Neuroscience)
Positron Emission Tomography - This involves introducing a low activity, short-lasting radioactive label to compounds like glucose or oxygen in the brain. The radioactive labels decay in a characteristic way, giving off sub-atomic particles (positrons). By surrounding the subject's head with a detector array, connected to a suitable computer, it is possible to build up images of the brain showing different levels of radioactivity, and therefore, cortical activity.
Problems: Expense, inaccessibility, lack of temporal (40 seconds) and spatial (1 cm) resolution.
fMRI (Neuroscience)
Functional Magnetic Resonance Imaging - This measures changes in oxygen levels in the brain which is an indicator of blood flow, a property of cortical activity. The amount of oxygen carried in the blood affects the blood’s magnetic properties. fMRI can detect functionally induced changes in blood oxygenation with a spatial resolution of about 2mm.
This technique builds on the foundation laid by MRI scanning, which is similar to CT in only measuring the structure of the brain. Functional MRI uses contrasts that are weighted for the detection of blood oxygenation: the assumption here is that activation in brain regions necessitates the delivery of more blood than would be required if the brain region were inactive. Thus, findings you typically see reported are the result of subtraction contrasts, usually (active condition) – (control condition). Subtraction contrasts, such as those shown on the right, give the impression that very little activation is occurring in the brain at any one time, but this is misleading. In fact, all of the brain is active all of the time. The differences are in the degree of activation.
The awareness that brain activation is always present has to a new understanding of functional brain systems known as functional connectivity networks or resting connectivity networks. Using functional connectivity analysis, it is possible to scan participants when they are not actively engaging in any cognitive tasks, and to build a picture of brain regions whose activation increases and decreases in concert over a prolonged time period (~10 seconds from peak to peak). Functional connectivity networks, such as those shown on the left (blue and red colours denote different functional networks) give a good idea of the way activation patterns persist across different regions of the brain even when people aren’t actively ‘thinking’. This suggests that the brain maintains a ‘holding pattern’ of activation that groups regions that are typically recruited by various cognitive tasks (e.g. memory systems, control systems etc.)
Problems: fMRI is expensive, and has poor temporal resolution (whole brain images can typically only be collected every 2 seconds).
EEG (Neuroscience)
Electroencephologram and Event-related Potentials (ERPs). Electro-chemical signals are the basis of communication between nerve cells and these can be recorded at the scalp. The first recordings from the human brain were published in 1924.
The amplitude of normal EEG varies approximately between -100 and + 100 microvolts. The EEG recorded at the scalp is only a gross measure of the activity of large numbers of neurones but several frequency patterns may be distinguished in the EEG. Waves which occur in normal EEG include the delta rhythm at 1-4 Hz, the Theta rhythm at 4-8 Hz, the alpha rhythm at 8-12 Hz and the Beta rhythm at 13-20 Hz. Two measurements are commonly used to analyze an EEG record. The amplitude, or size of the waves, and the number of waves per second.
Broadly speaking the more relaxed a person is the greater the amplitude and the lower the frequency of the waves. The lower the amplitude and the greater the frequency then the more likely it is that the person is in an excited state.
About 20 electrodes are normally applied placed according to the '10/20' system developed by Jasper (1958). In this system each electrode is placed in terms of its proximity to particular brain regions - Frontal, Central, Temporal, Parietal and Occipital. Sites are given an odd number when on the left side of the head and an even number on the right, and midline electrodes are labeled 'z'. The most common form of ERP recording is made between a scalp electrode located at a site of interest and a reference electrode usually placed at a site which is relatively uninfluenced by the electrical activity of experimental interest.
Recordings are based on the difference in voltage between each exploring electrode and the common reference electrode. Eye and jaw movements can cause fluctuating electrical fields across the scalp thus subjects are requested to remain still and to minimise eye blinks/movements. Eye movement is also recorded with the EEG so that trials on which there are gross movements can be eliminated or corrected from the analysis.
Current systems can record from up to 128 sites simultaneously using a 'geodesic dense array sensor net'. By making maps of the ERPs at different times after the stimulus event the relative times at which certain brain areas become active in processing information can be determined.
Problems: Spatial resolution is poor in comparison to other imaging techniques.


BRAIN GAMES/EXTRAS
